Richard Harland
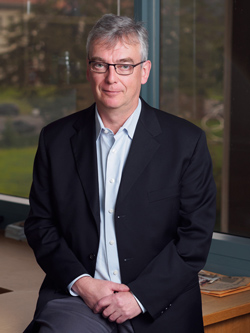
Dean of Biological Sciences, C.H. Li Distinguished Professor, and Professor of Genetics, Genomics, Evolution, and Development
Lab Homepage: http://mcb.berkeley.edu/labs/harland/Research Interests
The objective of our work is to understand early vertebrate development at the molecular level. We study this problem in both the amphibian Xenopus, and in the mouse. Xenopus embryos are large and easily manipulated, so that the function of various macromolecules, such as RNA and protein, can be assayed by microinjection into living embryos. Functional assays in Xenopus can then be complemented by genetic knockouts in the mouse, to gain fuller understanding of the normal requirements for gene action in the developing embryo.
Current Projects
Axis formation, induction and patterning of the Neural Plate: The dorsal-ventral organization of the gastrula arises from a series of symmetry breaking steps, including the activation of early Wnt/beta catenin signaling, which synergizes with Nodal/Smad2 signaling to generate Spemann’s Organizer. Where Smad2 and beta-catenin signals overlap, the Organizer genes are expressed, and several of these are BMP antagonists, which prevent ventral BMP signals from inducing epidermis and ventral mesoderm, and allow, by default, the formation of the nervous system and dorsal mesoderm. At the same time the nervous system becomes patterned in the anterior posterior axis to form brain and spinal cord, with the default fate being brain, and spinal cord being induced by a combination of Fibroblast Growth Factor and a re-use of Wnt/beta catenin signaling (1).
To identify new signaling mechanisms, we developed methods to screen libraries of mRNAs to identify potent bioactivities, such as molecules that dorsalize the embryo (2). We then use explants to study how these signals determine the state of tissues at different times- when and how do these tissues become specified to form epidermis and nervous system, for example, in response to these signals; we also determine the biochemical mechanism for the activity. Noggin is one such molecule, which binds tightly to the BMPs and prevents them from signaling (3).
Shape changes in development: In addition to the molecules that determine cell fates, we are also interested in the cellular mechanisms that enable embryonic tissues to form the shape and organization of the tadpole. We showed that the Planar Cell Polarity pathway, originally studied in Drosophila, is also used in the frog gastrula, when the simple organization of the cleaving embryo rearranges to form the three germ layers of ectoderm, mesoderm and endoderm (4). This pathway continues to operate to elongate the tadpole, and along with the rolling up of the neural plate (5), is an important part of extending and closing the neural tube.
Genetics and Genomics of Xenopus: While Xenopus laevis, the South African Clawed frog, remains an ideal model to combine experimental manipulations of tissues, because of the large size of the embryo, and tolerance to injections and microsurgery, we have complemented this work with the use of the Western Clawed Frog, Xenopus tropicalis, which has a simpler, diploid genome, smaller size and more rapid development, all of which enable us to raise frogs quickly to exploit manipulations of the genes and genomes, in both forward and reverse genetic screens (6-8).
Both X. laevis and X. tropicalis are well suited to genome-wide approaches, and because of the large size of the embryo, we can isolate sufficient RNAs or proteins from just a few embryos for systems-level analysis. To facilitate this approach, we have collaborated with our colleague Dan Rokhsar and others to sequence and assemble the genome of X. tropicalis (9), and are completing the assembly of the X. laevis genome; we are continuing this approach with other frogs, so that we can contribute well-annotated and complete genomes for work with different frogs. We have used these assembled genomes to study the contribution of individual splicing factors to the organized maturation of messenger RNAs (10), and in other current projects, are using the assembled genomes to study the activation of gene expression by Wnt/beta catenin signaling during formation of the spinal cord and neural crest (11).
BMP antagonists in Mouse Development: To understand the role of BMP signaling and its attenuation in mammalian development, we have made mutants in BMP antagonists in the mouse. BMP signaling is used in many contexts, and the potent activity must be blocked in many developmental events to permit normal development (12). We have recently been most interested in the activity of overlapping BMP antagonists in development of the axial skeleton, where BMP signaling must first be prevented to allow formation of the skeletal precursors, and then permitted to permit the differentiation of these precursors (13).
BMP signaling must also be attenuated in the formation of the gut, where excess BMP signaling eliminated cells that control the smooth muscle activity and in a separate process prevents proper maturation of the stem cells of the intestinal crypts.
Selected Publications
1. R. Harland, J. Gerhart, Formation and function of Spemann's organizer. Annu Rev Cell Dev Biol 13, 611 (1997).
2. W. C. Smith, R. M. Harland, Expression cloning of noggin, a new dorsalizing factor localized to the Spemann organizer in Xenopus embryos. Cell 70, 829 (Sep 4, 1992).
3. L. B. Zimmerman, J. M. De Jesus-Escobar, R. M. Harland, The Spemann organizer signal noggin binds and inactivates bone morphogenetic protein 4. Cell 86, 599 (Aug 23, 1996).
4. J. B. Wallingford et al., Dishevelled controls cell polarity during Xenopus gastrulation. Nature 405, 81 (May 4, 2000).
5. S. L. Haigo, J. D. Hildebrand, R. M. Harland, J. B. Wallingford, Shroom induces apical constriction and is required for hingepoint formation during neural tube closure. Curr Biol 13, 2125 (Dec 16, 2003).
6. H. A. Chung, S. Medina-Ruiz, R. M. Harland, Sp8 regulates inner ear development. Proc Natl Acad Sci U S A, (Apr 10, 2014).
7. J. J. Young et al., Efficient targeted gene disruption in the soma and germ line of the frog Xenopus tropicalis using engineered zinc-finger nucleases. Proc Natl Acad Sci U S A 108, 7052 (Apr 26, 2011).
8. R. M. Harland, R. M. Grainger, Xenopus research: metamorphosed by genetics and genomics. Trends Genet 27, 507 (Dec, 2011).
9. U. Hellsten et al., The genome of the Western clawed frog Xenopus tropicalis. Science 328, 633 (Apr 30, 2010).
10. D. S. Dichmann, R. M. Harland, fus/TLS orchestrates splicing of developmental regulators during gastrulation. Genes Dev 26, 1351 (Jun 15, 2012).
11. J. J. Young, R. A. Kjolby, N. R. Kong, S. D. Monica, R. M. Harland, Spalt-like 4 promotes posterior neural fates via repression of pou5f3 family members in Xenopus. Development 141, 1683 (Apr, 2014).
12. L. J. Brunet, J. A. McMahon, A. P. McMahon, R. M. Harland, Noggin, cartilage morphogenesis, and joint formation in the mammalian skeleton. Science 280, 1455 (May 29, 1998).
13. D. A. Stafford, S. D. Monica, R. M. Harland, Follistatin interacts with Noggin in the development of the axial skeleton. Mech Dev, (Oct 25, 2013).
Photo credit: Mark Hanson at Mark Joseph Studios.
Last Updated 2014-04-28