James Hurley
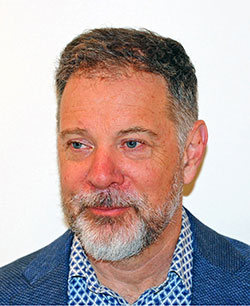
Kirsch Springer Chair in Biological Sciences and Professor of Cell Biology, Development and Physiology
Lab Homepage: http://membrane.berkeley.edu/Research Interests
The interplay between proteins and membrane lipids is central to almost every aspect of cell biology, and profoundly important to understanding disease mechansims, from viral infection to neurodegeneration. This laboratory is interested in fundamental questions of how the interactions between proteins and membranes determine cell and organelle shape and the evolution of shape over time, how protein-membrane interactions turn on and off the signals that control essential cell processes, and how pathogens such as HIV-1 subvert and co-opt these interactions. The roots of our research program are in the use of structural approaches, including x-ray crystallography, hydrogen-deuterium exchange, cryo-electron microscopy, and cryo-electron tomography, to reveal the structures and dynamics of membrane-interacting proteins across multiple resolution and scale regimes. The reconstitution and biophysical study of membrane remodeling pathways in synthetic membrane systems has taken on equal importance in the lab.
Current Projects
The ESCRT System
The ESCRT complexes catalyze one of the most unusual and mysterious membrane remodeling reactions in the cell. ESCRTs are responsible for the formation of multivesicular bodies (MVBs) in eukaryotic cells, remarkable structures that are required for transport of receptors and other membrane proteins to the lysosome. ESCRTs promote the budding of membrane away from cytosol, in other words, in the opposite direction from clathrin and other vesicle coats. The ESCRTs appear to promote this unusual mode of budding by forming an assembly at the bud neck, so as to temporarily stabilize the bud. The ESCRTs then go on to sever the membrane neck so as to release the bud. This membrane severing reaction is essential not only for MVB biogenesis, but also for the final severing of the narrow membrane neck separating two daughter cells during cytokinesis, for the release of microvesicles from the plasma membrane, and for the release of HIV-1 and a number of other viruses from the plasma membrane of infected cells. Our group has been developing a structural hypothesis for this unique membrane budding and severing pathway. The current focus of the lab is to understand at the structural and mechanistic level how the ESCRTs are co-opted by HIV-1 so as to release nascent virions from infected cells. We are also exploring how ESCRTs carry out lysosomal and plasma membrane repair.
Autophagy
Autophagy is a form of cellular self-cannibalism that appears at first glance to be bizarre and harmful. Yet autophagy is conserved throughout eukaryotes and profoundly important for cellular health and survival. Defects in autophagy in single-celled organisms impair survival under starvation and stress. In humans, dysfunction in autophagy is linked to most of the major ailments of the developed world, including neurodegenerative diseases, cardiovascular disease, and cancer. Autophagy proceeds by the engulfment of cytosol, organelles, and inclusions by a growing double-membraned sheet. The double membrane becomes sealed and fuses with the lysosome (or vacuole in yeast), and its contents are hydrolyzed and recycled. Many, if not most, of the proteins required for autophagy have been identified, yet little is known about how they work with each other and with membranes to carry out this remarkable pathway. Our laboratory has characterized the structures of the ULK1 and class III PI 3-kinase complexes, which are responsible for initiating autophagy. We have also carried out extensive reconstitutions of the process using purified components. The group now has a strong focus on building up an integrated structural model for autophagosome formation in the context of damaged-induced mitophagy in neurons as it related to Parkinson's Disease.
Cargo sorting into coated vesicles: host function and viral co-optation
In comparison to the counter-intuitive and poorly understood ESCRT and autophagy pathways, far more is known about coated vesicle formation at the level of molecular mechanism. Because so much of the groundwork has already been done in this field, we can ask subtle and sophisticated questions about the structural mechanisms of regulation and the redirection of basic functions by pathogens. Cargo adaptors typically link membrane proteins to coat components. We have been focusing on the AP family of heterotetrameric adaptor complexes. We were recently able to resolve a question that had stood in this field for 20 years: how the small GTPase Arf1 activates AP complexes for cargo binding. Other current efforts focus on the multiple ways that HIV-1 subverts the normal functions of the AP complexes, redirecting them to undermine the cell’s own defenses. HIV-1:host interactions of this type are attractive as novel drug targets, because host proteins are not subject to the same rapid mutation as HIV proteins, and are therefore less susceptible to the emergence of drug resistance.
Lysosomes
We are using cryo-EM and biochemisty to understand how the FLCN-FNIP and C9orf72-SMCR8 complexes regulate signaling at the lysosomal membrane. This work uncovered the existence of the Lysosomal Folliculin Complex (LFC), which antagonizes the RagC GAP activity of FLCN in amino acid starvation, and showed how SLC38A9 breaks up the LFC upon refeeding. Our C9orf72 work led to the discovery that the C9orf72-SMCR8 complex is an ARF GAP, which may have implication for developing therapeutics for Frontotemporal Degeneration (FTD) and Amyotrophic Lateral Sclerosis (ALS).
Selected Publications
Morris, K. L, Buffalo, C., Sturzel, C., Heusinger, E., Kirchhoff, F., Ren, X. and Hurley, J. H. HIV-1 Nefs are Cargo-Sensitive AP-1 Trimerization Switches in Tetherin and MHC-I Downregulation. Cell 174, 659-671 (2018).
Schöneberg, J., Remec Pavlin, M., Yan, S., Righini, M., Lee, I.-H., Carlson, L. A., Bahrami, A. H., Goldman, D. H., Ren, X., Hummer, G., Bustamante, C. and Hurley, J. H. ATP-dependent force generation and membrane scission by ESCRT-III and Vps4. Science, 362, 1423-1428 (2018).
Buffalo, C. Z., Sturzel, C., Heusinger, E., Kmiec, D., Kirchhoff, F., Hurley, J. H. *, and Ren, X. Structural Basis for Tetherin Antagonism as a Barrier to Zoonotic Lentiviral Transmission. Cell Host & Microbe 26, 359-368 (2019). Highlighted in a Preview in Cell Host & Microbe 26, 299-300 (2019).
Flower, T. G., Takahashi, Y., Hudait, A., Rose, K., Tjahjono, N., Pak, A. J., Yokom, A. L., Liang, X., Wang, H.-G., Bouamr, F., Voth, G. A., and Hurley, J. H. A Helical Assembly of Human ESCRT-I Scaffolds Reverse-Topology Membrane Scission. Nat. Struct. Mol. Biol. doi.org/10.1038/s41594-020-0426-4 (2020).
Chang, C., Young, L. N., Morris, K. L., von Bülow, S., Schöneberg, J., Yamamoto-Imoto, H., Oe, Y., Yamamoto, K., Nakamura, S., Stjepanovic, G., Hummer, G., Yoshimori, T., and Hurley, J. H. Bidirectional Control of Autophagy by BECN1 BARA Domain Dynamics. Mol. Cell 73, 339-353 (2019).
Shi, X., Yokom, A. L., Wang, C., Young, L. N., Youle, R. J., and Hurley, J. H. ULK Complex Organization in Autophagy by a C-shaped FIP200 N-Terminal Domain Dimer. J. Cell. Biol., 219, e201911047. doi: 10.1083/jcb.201911047(2020).
Shi, X., Chang, C., Yokom, A. L. and Hurley, J. H. The Autophagy Adaptor NDP52 and the FIP200 Coiled-Coil Allosterically Activate ULK1 Complex Membrane Recruitment. eLifedoi: 10.7554/eLife.59099 (2020).
Lawrence, R. E., Fromm, S. A., Fu, Y., Yokom, A. L., Kim, D. J., Thelen, A. M., Young, L. N., Lim, C. Y., Samelson, A. J., Hurley, J. H.*, and Zoncu, R. Structural Mechanism of a Rag GTPase Activation Checkpoint by the Lysosomal Folliculin Complex. Science 366, 971-977 (2019). *co-corresponding author.
Su, M.-Y., Fromm, S. A., Zoncu, R. and Hurley, J. H. Structure of the C9orf72 Arf GAP complex haploinsufficient in ALS and FTD. Nature, 585, 251-255. (2020).
Fromm, S.A., Lawrence, R. E. and Hurley, J. H. Structural Mechanism for Amino Acid-Dependent Rag GTPase Nucleotide State Switching by SLC38A9. Nat. Struct. Mol. Biol. doi: 10.1038/s41594-020-0490-9 (2020).
Photo credit: Calin Hurley.
Last Updated 2021-07-05