Doug Koshland
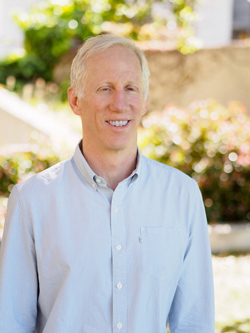
Richard and Rhoda Goldman Distinguished Chair in the Biological Sciences & Professor of Genetics, Genomics, Evolution, and Development*
**And Affiliate, Division of Cell and Developmental Biology
Research Interests
My laboratory uses budding yeast to study fundamental processes in cell biology. Our approach has been to develop novel genetic reagents and cell biological methods to analyze these complex processes in vivo, coupled with the development and exploitation of in vitro assays to reveal underlying molecular mechanisms. Together these approaches have allowed us to make significant contributions to following three areas of research.
- Higher Order Chromosome Structure is important for the integrity, transmission and expression of chromosomes. The molecular bases for the formation, maintenance and disassembly of higher order chromosome organization are major unsolved problems in cell biology.
- The Maintenance of Genome Integrity (chromosome number and integrity) is critical to prevent cell inviability, inherited disorders and disease. A major threat to genome integrity is breaks in the DNA that can be misrepaired to cause gross chromosomal rearrangements (GCR) such as translocations, internal deletions or terminal truncations. While the cause of DSBs and their repair have been studied intensively, major new discoveries will be required to explain the massive rearrangement of chromosomes associated with cancer.
Current Projects
Chromosome Structure
Higher-order organization of chromosomes is essential for chromosome segregation, DNA damage repair, and the regulation of gene expression. From bacterial to human cells, higher-order chromosome structure is mediated by a conserved family of protein complexes named after their shared SMC (Structural Maintenance of Chromosomes) subunits. Mutations in SMC subunits help drive cancer and cause age-dependent congenital disabilities and developmental disorders. SMC complexes have two activities that structure chromosomes. First, they tether together two regions of DNA, either within a single DNA molecule or between DNA molecules. Second, by combining this tethering activity with their ability to translocate along DNA, SMC complexes can also extrude DNA loops (loop extrusion) in vivo and in vitro. Key questions about the biological and molecular functions of these remarkable complexes remain unanswered.
The SMC complex called cohesin is essential for sister chromatid cohesion and condensation as well as playing critical roles in DNA repair and gene expression. During the last five years, my laboratory used existing and novel assays to reveal that cohesin is a much more complicated machine than previously proposed and elucidated key new features of its DNA binding, DNA tethering, in vivo confirmation and oligomerization, loop extrusion, and regulation.
The complexity of cohesin's biological and biochemical functions pose many important unanswered questions. What are the molecular activities that allow cohesin both to translocate along DNA and to tether two regions of DNA? What regulatory mechanism(s) designate subsets of cohesins for tethering and loop extrusion? Initially the primary biological function of loop extrusion was thought to be the control of gene expression. However, more recent studies from our lab and others suggest that the majority of loops formed by cohesin are likely involved in a different aspect of chromosome metabolism. What is this mysterious function? To answer these questions, my lab interrogates cohesin's biochemical and biological functions by exploiting our extensive mutant collection in cohesin and cohesin regulators along with novel in vivo and in vitro assays developed by us and others.
Selected Publications
Çamdere, G.Ö., Carlborg, K.K., and Koshland, D. (2018). Intermediate step of cohesin’s ATPase cycle allows cohesin to entrap DNA. Proceedings of the National Academy of Sciences 4, 201807213.
Photo Credit: Mark Hanson of Mark Joseph Studios
Last Updated 2024-08-25