John G. Flannery
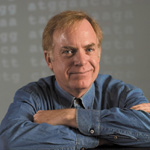
Professor of Molecular Therapeutics
Lab Homepage: http://mcb.berkeley.edu/labs/flannery/Research Interests
Molecular Biology and Gene Therapies for Inherited Retinal Degenerations
Inherited forms of retinal degeneration, which afflict 1 in 3000 people worldwide, arise primarily from mutations in transcripts expressed in rod and cone photoreceptors and retinal pigment epithelial cells. The outer retina is therefore the primary target for ocular gene therapies. Photoreceptor degeneration is a very genetically heterogeneous disorder; with mutations in over 200 loci identified. Our laboratory focuses on understanding the genetic and mechanistic underpinning of photoreceptor degeneration, and developing rational therapies for these blinding conditions. The genetic and biochemical diversity of photoreceptor degnereration presents major challenges for therapy as there are many pathways to cell death.
Gene therapy has great potential for treating retinal diseases including glaucoma, age-related macular degeneration, and inherited photoreceptor diseases. To date, most gene therapies have targeted monogenic recessive retinal diseases and employed viral vectors to transfer a 'normal ' copy of the mutated gene to the affected cell. We are currently developing animal models of inherited retinal diseases to study the disease processes. In parallel, we are designing viral mediated therapies for autosomal dominant and recessive retinal degnenerations.
Current Projects
The retina is a complex tissue in the back of the eye that contains the rod and cone photoreceptor cells. The photoreceptors connect to a network of retinal interneurons. The adjacent retinal pigment epithelium (RPE) supports many of the retina's metabolic functions. The retina is susceptible to a number of blinding diseases, such as age-related macular degeneration, diabetic retinopathy and other inherited retinal degenerations. The inherited retinal degenerations are typified by retinitis pigmentosa (RP), which results in blindness from destruction of photoreceptor cells, and the RPE. This group of conditions affects approximately 100,000 people in the United States. To date, more than 130 genes causing inherited retinopathies in humans have been identified. This makes it possible to identify the cause of RP in approximately 50 percent of patients and the cause of Usher syndrome in 75 percent of patients.
Gene identifications in humans have allowed us to examine the biochemical pathways in these diseases. In addition, gene identification in patients permits us to identify naturally occurring animal models or create new transgenic or knockout animal models with retinal degeneration due to defects in the gene homologs. In particular, we have the examined retinal degeneration in the naturally arising rd mouse strains (defects in the b-subunit of phosphodiesterase). We have also developed transgenic rats, expressing dominant rhodopsin mutations. Most recently we have developed a knockout mouse model of Usher syndrome type 3, which caused progressive blindness and deafness in patients. These animal models are the subject of study to determine the pathophysiological mechanisms whereby these gene defects lead to photoreceptor degeneration and hopefully will lead to pilot studies of novel therapies for retinal degeneration.
Development of effective treatments for retinal diseases.
With increasing insight into the molecular etiologies of several inherited retinal and macular dystrophies, studies from ours and many laboratories have defined several promising therapeutic strategies.
Current projects in our lab involve development of retinal cell specific viral vectors based upon lentivirus and adeno-associated viruses. In previous work, we have demonstrated significant slowing of photoreceptor degeneration in several animal models following gene transfer of neurotrophic agents. Another promising strategy for dominantly inherited retinal diseases involves directly targeting the mutant mRNA product using Talens, CRISPR, and siRNA constructs. For recessive null diseases, gene replacement is an option.
We find that gene therapy has vast potential for treating and potentially curing a number of inherited photoreceptor diseases. However, gene delivery technologies require significant improvements in cellular targeting, efficiency, and safety before promising findings in animal studies are translated to the clinic. In particular, for retinal gene therapy it would be highly advantageous to transduce a single cell type that spans the entire retina after an intravitreal injection of a gene delivery vehicle for the subsequent secretion of a general neuroprotective factor throughout the retina. Unfortunately, there is no vector capable of efficiently infecting the cell type that meets these needs, Muller cells. Vectors based on adeno-associated virus (AAV) have proven themselves to be highly promising in numerous retinal disease models, but they are also incapable of Muller cell infection. Recently, we have developed novel lentiviral vectors with new properties, including altered receptor binding, which are capable of efficient Muller cell transduction. In parallel, the basic mechanisms of AAV transduction of Muller cells will be explored in order to develop new AAV pseudotypes capable of Muller cell transduction. The novel approaches developed in this work will have general impact for the molecular engineering of enhanced viral gene delivery vehicles, and future work will focus on testing these vectors in an animal model of retinal disease.
Selected Publications
1. Byrne, L.C. et al. In vivo-directed evolution of adeno-associated virus in the primate retina. JCI Insight 5 (2020).
2. Kim, J. et al. Oblique-plane single-molecule localization microscopy for tissues and small intact animals. Nat Methods 16, 853-857 (2019).
3. Berry, M.H. et al. Restoration of high-sensitivity and adapting vision with a cone opsin. Nat Commun 10, 1221 (2019).
4. LaVail, M.M. et al. Phenotypic characterization of P23H and S334ter rhodopsin transgenic rat models of inherited retinal degeneration. Exp Eye Res 167, 56-90 (2018).
5. Hardcastle, A.J. et al. Translational Retinal Research and Therapies. Transl Vis Sci Technol 7, 8 (2018).
6. Gaub, B.M. et al. Optogenetic Retinal Gene Therapy with the Light Gated GPCR Vertebrate Rhodopsin. Methods Mol Biol 1715, 177-189 (2018).
7. Fortuny, C. & Flannery, J.G. Mutation-Independent Gene Therapies for Rod-Cone Dystrophies. Adv Exp Med Biol 1074, 75-81 (2018).
8.Elsaeidi, F. et al. Notch Suppression Collaborates with Ascl1 and Lin28 to Unleash a Regenerative Response in Fish Retina, But Not in Mice. J Neurosci 38, 2246-2261 (2018).
9.Duncan, J.L. et al. Inherited Retinal Degenerations: Current Landscape and Knowledge Gaps. Transl Vis Sci Technol 7, 6 (2018).
10.Day, T.P., Byrne, L.C., Flannery, J.G. & Schaffer, D.V. Screening for Neutralizing Antibodies Against Natural and Engineered AAV Capsids in Nonhuman Primate Retinas. Methods Mol Biol 1715, 239-249 (2018).
11. Baker, C.K. & Flannery, J.G. Innovative Optogenetic Strategies for Vision Restoration. Front Cell Neurosci 12, 316 (2018).
12. Hickey, D.G. et al. Tropism of engineered and evolved recombinant AAV serotypes in the rd1 mouse and ex vivo primate retina. Gene Ther 24, 787-800 (2017).
13. Yao, K. et al. Wnt Regulates Proliferation and Neurogenic Potential of Muller Glial Cells via a Lin28/let-7 miRNA-Dependent Pathway in Adult Mammalian Retinas. Cell Rep 17, 165-178 (2016).
14. Shen, S.Q. et al. Massively parallel cis-regulatory analysis in the mammalian central nervous system. Genome Res 26, 238-255 (2016).
15. Zawadzki, R.J. et al. Adaptive-optics SLO imaging combined with widefield OCT and SLO enables precise 3D localization of fluorescent cells in the mouse retina. Biomed Opt Express 6, 2191-2210 (2015).
16. Xue, Y. et al. CRALBP supports the mammalian retinal visual cycle and cone vision. J Clin Invest 125, 727-738 (2015).
17.Thompson, D.A. et al. Advancing therapeutic strategies for inherited retinal degeneration: recommendations from the Monaciano Symposium. Invest Ophthalmol Vis Sci 56, 918-931 (2015).
18. Rosa, J.M. et al. Neuron-glia signaling in developing retina mediated by neurotransmitter spillover. Elife 4 (2015).
19. Pellissier, L.P. et al. Gene therapy into photoreceptors and Muller glial cells restores retinal structure and function in CRB1 retinitis pigmentosa mouse models. Hum Mol Genet 24, 3104-3118 (2015).
20. Kotterman, M.A. et al. Antibody neutralization poses a barrier to intravitreal adeno-associated viral vector gene delivery to non-human primates. Gene Ther 22, 116-126 (2015).
21. Gaub, B.M., Berry, M.H., Holt, A.E., Isacoff, E.Y. & Flannery, J.G. Optogenetic Vision Restoration Using Rhodopsin for Enhanced Sensitivity. Mol Ther 23, 1562-1571 (2015).
22. Byrne, L.C., Lin, Y.J., Lee, T., Schaffer, D.V. & Flannery, J.G. The expression pattern of systemically injected AAV9 in the developing mouse retina is determined by age. Mol Ther 23, 290-296 (2015).
23.Byrne, L.C. et al. Viral-mediated RdCVF and RdCVFL expression protects cone and rod photoreceptors in retinal degeneration. J Clin Invest 125, 105-116 (2015).
24. Yin, L. et al. Imaging light responses of foveal ganglion cells in the living macaque eye. J Neurosci 34, 6596-6605 (2014).
25. Vlasits, A.L. et al. Visual stimulation switches the polarity of excitatory input to starburst amacrine cells. Neuron 83, 1172-1184 (2014).
26. Vacca, O. et al. AAV-mediated gene delivery in Dp71-null mouse model with compromised barriers. Glia 62, 468-476 (2014).
27.Pellissier, L.P. et al. Specific tools for targeting and expression in Muller glial cells. Mol Ther Methods Clin Dev 1, 14009 (2014).
28. Gaub, B.M. et al. Restoration of visual function by expression of a light-gated mammalian ion channel in retinal ganglion cells or ON-bipolar cells. Proc Natl Acad Sci U S A 111, E5574-5583 (2014).
29. Day, T.P., Byrne, L.C., Schaffer, D.V. & Flannery, J.G. Advances in AAV vector development for gene therapy in the retina. Adv Exp Med Biol 801, 687-693 (2014).
30. Byrne, L.C. et al. Retinoschisin gene therapy in photoreceptors, Muller glia or all retinal cells in the Rs1h-/- mouse. Gene Ther 21, 585-592 (2014).
31. Alves, C.H. et al. Targeted ablation of Crb2 in photoreceptor cells induces retinitis pigmentosa. Hum Mol Genet 23, 3384-3401 (2014).
Last Updated 2020-09-11