Jamie H. D. Cate
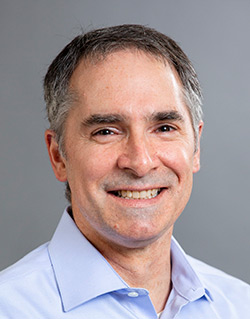
Professor of Biochemistry, Biophysics and Structural Biology*
*and of Chemistry
Research Interests
Protein synthesis by the "universal translator" in biology
The Cate Lab explores how genes are put into action by translation. Translation is the universal process of protein synthesis, in which the ribosome translates the four-letter genetic code in messenger RNA into the twenty-letter code of proteins. The lab also works on strategies for making new sequence defined polymers using engineered ribosomes.
Regulating human translation
Human bodies are quite complex, with many different cell types and tissues, but only one genome. To put information in our genome into action, the different steps of gene expression must be regulated to lay out our body plan, and to maintain it. We are beginning to appreciate that regulating when and where proteins are made from the messenger RNA (mRNA) copies of our genes is critical for us to develop and stay healthy.
Proteins are polymers, which means protein synthesis–or translation–happens in three overall steps: initiation, elongation, and termination. In all of life, translation initiation is heavily regulated. That's probably because it's better not to start making a protein until it's needed, rather than stopping in the middle of making it. In humans, translation initiation involves many general translation factors, proteins and protein complexes called eukaryotic translation initiation factors or eIFs. We have focused on eIF3, because its large size remains a mystery.
Human eIF3 is targeted by viruses like the hepatitis C virus that highjack translation for their own ends. We think that these viruses are tapping in to specific ways that eIF3 is used in normal human biology. With this idea in mind, we recently discovered that eIF3 is more than a general translation initiation factor. It can activate or repress translation of specific mRNAs that control how cells grow and divide. Notably, a number of these mRNAs encode key proteins involved in cancer. We're now working to understand how eIF3 binds these mRNAs to turn them on or off. We know that RNA structure is likely involved, rather than just a linear sequence pattern. We're also interested to find out if eIF3 regulates other mRNAs in different kinds of cells, like T cells and neural progenitor cells. To answer these questions, we are using systems biology, biochemistry and structural biology including cryo-electron microscopy (cryo-EM).
Engineering ribosomes
We are working with the Center for Genetically Encoded Materials to discover ways to engineer bacterial ribosomes to make new kinds of polymers–sequence defined polymers encoded by mRNA–rather than proteins. We study how ribosome structure and function can be altered to enable new polymerization chemistry in the ribosome. We are interested in designing screens for new activity, probing mutant ribosomes in vitro and with cryo-EM, and in gaining a better understanding of how the ribosome can be engineered.
Selected Publications
Majumdar C, Walker JA, Francis MB, Schepartz A, Cate JHD (2023) Aminobenzoic acid derivatives obstruct induced fit in the catalytic center of the ribosome. BioRxiv
Nissley AJ, Penev PI, Watson ZL, Banfield JF, Cate JHD (2023) Rare Ribosomal RNA Sequences from Archaea Stabilize the Bacterial Ribosome. Nucleic Acids Res. doi: 10.1093/nar/gkac1273. PMID: 36660825.
De Silva D, Ferguson L, Smith BE, Chin GE, Apathy RA, Roth TL, Kudla M, Marson A, Ingolia NT, Cate JHD. (2021) Robust T cell activation requires an eIF3-driven burst in T cell receptor translation. eLife 10, e74272. PMID: 34970966.
Li W, Chang ST, Ward FR, Cate JHD. (2020) Selective inhibition of human translation termination by a drug-like compound. Nat. Commun. 11, 4941. PMID: 33009412, PMCID: PMC7532171.
Tharp JM, Ad O, Amikura K, Ward FR, Garcia EM, Cate JHD, Schepartz A, Söll D. (2019) Initiation of protein synthesis with non-canonical amino acids in vivo. Angew Chem Int Ed Engl. Dec 11. doi: 10.1002/anie.201914671. [Epub ahead of print] PMID: 31828898.
Ward FR, Watson ZL, Ad O, Schepartz A, Cate JHD. (2019) Defects in the Assembly of Ribosomes Selected for β-Amino Acid Incorporation. Biochemistry. 58,4494-4504. doi: 10.1021/acs.biochem.9b00746. PMID: 31607123.
Pulos-Holmes MC, Srole DN, Juarez MG, Lee AS, McSwiggen DT, Ingolia NT, Cate JH. (2019) Repression of ferritin light chain translation by human eIF3. Elife. 8. pii: e48193. doi: 10.7554/eLife.48193. PMID: 31414986; PMCID: PMC672179.
Li W, Ward FR, McClure KF, Chang ST, Montabana E, Liras S, Dullea RG, Cate JHD. (2019) Structural basis for selective stalling of human ribosome nascent chain complexes by a drug-like molecule. Nat Struct Mol Biol. 26(6):501-509.doi: 10.1038/s41594-019-0236-8. PMID: 31160784.
Liaud N, Horlbeck MA, Gilbert LA, Gjoni K, Weissman JS, Cate JHD. (2019) Cellular response to small molecules that selectively stall protein synthesis by the ribosome. PLoS Genet. 15(3):e1008057. doi:10.1371/journal.pgen.1008057. PMID: 30875366; PMCID: PMC6436758.
Lintner NG, McClure KF, Petersen D, Londregan AT, Piotrowski DW, Wei L, Xiao J, Bolt M, Loria PM, Maguire B, Geoghegan KF, Huang A, Rolph T, Liras S, Doudna JA, Dullea RG†, Cate JHD† (2017) Selective stalling of human translation through small molecule engagement of the ribosome nascent chain. PLoS Biol., 15, e2001882. [†Corresponding authors.], PMID: 28323820. PMCID: PMC5360235.
Last Updated 2023-02-01