Ehud Isacoff
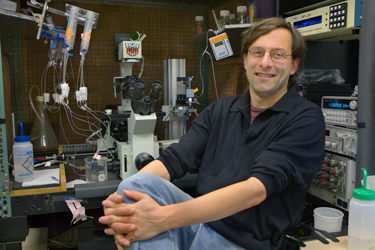
Professor of Biochemistry, Biophysics and Structural Biology
Lab Homepage: https://mcb.berkeley.edu/labs/isacoff/Research Interests
We work on the mechanisms of function of ion channels, neurotransmitter receptors and voltage-gated enzymes as well as on synaptic transmission, modulation and homeostatic plasticity. To enable these studies, we developed ensemble and single molecule optical methods that reveal conformational rearrangements in real time in channels, receptors and enzymes, chemical optogenetics that make it possible to activate or block channels and GPCRs, and super-resolution methods for quantal imaging of synaptic transmission. We apply these approaches to study molecular mechanisms of synaptic signaling proteins in isolation, in cells and in neural circuits. We are also them in effort to compensate for selective loss of dopamine signaling in models of Parkinson’s Disease and in drug discovery for depression and psychosis.
Current Projects
A) Allosteric control of channel and enzyme gates. Ion channels, GPCRs and enzymes respond to physiological signals by opening and closing molecular gates that control the flow of ions across the membrane, form and deform the G protein interaction site, and regulate access to a catalytic site. We combine electrophysiology, spectroscopy and molecular dynamics simulation to detect conformational changes in the moving parts of these membrane proteins during their functional transitions to understand the mechanism of coupling of sensor domains to gates and to one another, and thus to investigate the mechanism of allosteric control of gating. The work began with the development of VCF to study voltage sensing in K+ channels and is now focused on how the conformational changes of a voltage sensor can control “gating” of a catalytic site in a voltage sensing phosphatase as well as in single molecule imaging in live cell membranes and on isolated proteins on ionotropic (especially NMDA receptors) and metabotropic glutamate receptors to understand how multiple domains in multiple subunits allosterically control the functional output of a pore or G protein binding site and how these processes are regulated at synapses.
B) Synaptic function and plasticity. Activity-dependent and independent mechanisms regulate synaptic size and strength to ensure a balance between excitation and inhibition of inputs and between input and output. This appears to be accomplished by integrated postsynaptic adjustment of synaptic receptors and retrograde signaling back to the presynaptic cell to adjust neurotransmitter release. The molecular mechanisms underlying these processes are not known. We are studying these processes in dissociated mouse brain neurons and brain slice and at the model glutamatergic synapse of the Drosophila neuromuscular junction. We are using two tools developed in the lab. The first is a postsynaptic density targeted calcium sensor, which exclusively detects calcium influx through glutamate receptor-channels thereby making it possible to image synaptic transmission at quantal resolution. We recently developed super-resolution quantal (QuaSOR) analytic methods that make it possible to image transmission at hundreds of identified synapses at a time to define the molecular mechanisms that control transmitter release and plasticity in Drosophila and mammals. The second method involves light-controlled liganding of glutamate receptors (C, below) to determine the activity dependence of the retrograde transmission.
C) Novel probes for the optical manipulation of neuronal signaling. Together with Richard Kramer and Dirk Trauner, we developed chemical optogenetics. Rather than over-ride normal cell signaling with light-activated excitatory or inhibitory channels from another organism, in chemical optogenetics we synthesize photoswitched ligands for native channels or receptors. These are opto-chemical toggles for ligands that act as pore blockers of ion channels or agonists, antagonists or allosteric modulators of neurotransmitter receptors. They turn on and off rapidly and reversibly in response to light and can be tethered selectively to the channel or receptor of interest or to a membrane anchoring protein whose expression is driven in genetically selected cells and transported to a select subcellular location to control local native receptors and channels. The approach provides control of synaptic signaling proteins with selectivity, speed and reversibility. Our targets have included potassium channels as well as glutamate and dopamine receptors. The light-driven signaling has millisecond temporal control, sub-micrometer spatial precision and can be combined with 3D holography to control synaptic transmission and modulation in ensembles of synapses and cells. The ability to control NMDA receptors and mGluRs provides access to the plasticity that mediates memory, while light-control of dopamine receptors opens the way to understand reward, attention and initiation of action, and may provide novel approaches to the treatment of movement, cognitive or psychiatric disorders associated with neurodegeneration or dysregulation of dopamine.
Selected Publications
- Larsson, H.P., Baker, O.S., Dhillon, D.S. and Isacoff, E.Y. (1996). Transmembrane movement of the Shaker K+ channel S4. Neuron 16, 387-397.
- Mannuzzu, L.M., Maronne, M.M. and Isacoff, E.Y. (1996). Direct physical measure of conformational rearrangement underlying potassium channel gating. Science 271, 213-16.
- Glauner, K.S., Mannuzzu, L.M., Gandhi, C.S. and Isacoff, E.Y. (1999). Spectroscopic mapping of voltage sensor movement in the Shaker potassium channel. Nature 402, 813-17.
- Tombola, F. Pathak, M., and Isacoff, E.Y. (2005). Voltage-sensing arginines in a potassium channel permeate and occlude cation-selective pores. Neuron 45, 379-88.
- Tombola, F. Pathak, M., Gorostiza, P. and Isacoff, E.Y (2007). The twisted ion-permeation pathway of a resting voltage-sensing domain. Nature 445, 546-9.
- Tombola, F., Ulbrich, M. and Isacoff, E.Y. (2008). The voltage-gated proton channel Hv1 has two pores each controlled by one voltage sensor. Neuron 58, 546-556.
- Wyart, C., Bene, F.D., Warp, E., Scott, E.K., Trauner, D., Baier, H. and Isacoff, E.Y. (2009). Optogenetic dissection of a behavioural module in the vertebrate spinal cord. Nature 461, 407-10.
- Del Bene, F., Wyart, C., Robles, E., Tran, A., Scott, E.K., Isacoff, E.Y. and Baier, H. (2010). Filtering of visual information in the tectum by an identified neural circuit. Science 330, 669-673.
- Kohout, S.C., Bell, S.C. Lui, L., Xu, Q., Minor Jr., D.L. and Isacoff, E.Y. (2010). Electro-chemical coupling in the voltage-dependent phosphatase Ci-VSP. Nature Chem. Bio. 6, 369-75.
- Janovjak, H., Szobota, S., Wyart, C., Trauner, D. and Isacoff, E.Y. (2010). A light-gated, potassium-selective glutamate receptor for the optical inhibition of neuronal firing. Nature Neuroscience 13, 1027-32.
- Berger, T.K. and Isacoff, E.Y. (2011). The pore of the voltage-gated proton channel. Neuron 72, 991-1000.
- Peled, E.S. and Isacoff, E.Y. (2011). Optical Quantal Analysis of Synaptic Transmission from Wildtype and Rab3-Mutant Drosophila Motor Axons. Nature Neurosci. 14, 519-26.
- Sandoz, G., Levitz, J., Kramer, R.H., and Isacoff, E.Y. (2012). Optical control of endogenous proteins with a Photoswitchable Conditional Subunit reveals a role for TREK1 in GABA(B) signaling. Neuron 74, 1005-14.
- Levitz, J., Pantoja, C., Gaub, B. Janovjak, H. Reiner, R., Hoagland, A., Schoppik, D., Kane, B., Stawski, P., Schier, A.F., Trauner, D., and Isacoff, E.Y. (2013). Optical Control of Metabotropic Glutamate Receptors. Nat. Neurosci. 16, 507-16.
- Vafabakhsh, R., Levitz, J. and Isacoff, E.Y. (2015). Conformational dynamics of a class C G protein-coupled receptor. Nature 524, 497-501.
- Grimm, S.S. and Isacoff, E.Y. (2016). Allosteric substrate switching in a voltage sensing lipid phosphatase. Nature Chemical Biology 12, 261-7.
- Berlin, S., Szobota, S., Reiner, A., Kienzler, M., Guyon, A., Trauner, D. and Isacoff, E.Y. (2016). A family of photoswitchable NMDA receptors. eLife 5, e12040.
- Levitz, J., Habrian, C., Bharill,S., Fu, Z., Vafabakhsh, R. and Isacoff, E.Y. (2016). Mechanism of assembly and cooperativity of homomeric and heteromeric metabotropic glutamate receptors. Neuron 92, 143-159.
- Pantoja, C., Hoagland, A., Carroll, E.C, Karalis, V., Conner, A. and Isacoff, E.Y. (2016). Neuromodulatory Regulation of Behavioral Individuality in Zebrafish. Neuron 91, 587-601
- Levitz, J., Broichhagen, J., Leippe, P., Konrad, D., Trauner, D. and Isacoff, E.Y. (2017). Dual optical control and mechanistic insights into photoswitchable group II and III metabotropic glutamate receptors. PNAS 114, E3546-E3554.
- Newman, Z.L., Hoagland, A., Aghi, K., Worden, K.L., Levy, S.L., Son, J.H., Lee, L.P. and Isacoff, E.Y. (2017). Input-specific plasticity and homeostasis at the Drosophila larval neuromuscular junction. Neuron 93, 1388-1404.
- Habrian, C., Levitz, JL., Vyklicky, V., Fu, Z., Hoagland, A., McCort-Tranchepain, I., Acher, F. and Isacoff, E.Y. (2019). Conformational pathway provides unique sensitivity to a synaptic mGluR. Nature Commun. 10, 5572. doi: 10.1038/s41467-019-13407-8.
- Donthamsetti, P., Winter, N., Hoagland, A., Stanley, C., Visel, M., Lammel, S., Trauner, D. and Isacoff, E.Y. (2021). Cell-specific molecular gating of a dopamine receptor. Nature Commun. 12, 4775. doi: 10.1038/s41467-021-25003-w.
- Vyklicky,V., Stanley, C., Habrian, C. and Ehud Y. Isacoff (2021). Conformational rearrangement of the NMDA receptor amino-terminal domain during activation and allosteric modulation. Nature Commun. 12, 2694.
- Newman, Z.L., Bakshinskaya, D., Schultz, R, Kenny, S.J., Moon, S., Aghi, K., Stanley, C., Marnani, N., Li, R., Bleier, J., Xu, K. and Isacoff, E.Y. (2022). Determinants of synapse diversity revealed by super-resolution quantal transmission and active zone imaging. Nature Commun. 13, 229. doi: 10.1038/s41467-021-27815-2.
- Winans, A.M., Friedmann, D., Stanley, C., Xiao, T., Liu,T-S., Chang, C.J., and Isacoff, E.Y. (2023). Ciliary localization of a light-activated neuronal GPCR shapes behavior. PNAS 120(43):e2311131120. doi: 10.1073/pnas.2311131120. Epub 2023 Oct 16.
- Habrian, C., Latorraca, N., Fu, Z., and Isacoff, E.Y. (2023). Homo- and hetero-dimeric subunit interactions set affinity and efficacy in metabotropic glutamate receptors. Nature Commun. 14, 8288. DOI 10.1038/s41467-023-44013-4.
- Kumar, K.K., Wang, H., Habrian, C., Latorraca, N.R., Xu, H., O’Brien, E.S., Zhang, C., Montabana, E., Koehl, A., Marqusee, S., Isacoff, E.Y., and Kobilka, B.K. (2024). Step-wise activation of a family C GPCR. Nature (in press). BioRxiv. https://www.biorxiv.org/content/10.1101/2023.08.29.555158v1.article-metrics
- Bleier, J., Ribeiro Furtado de Mendonca, P., Habrian, C., Stanley, C., Vyklicky, V. and Isacoff, E.Y. (2024). Conformational basis of subtype-specific allosteric control of NMDA receptor gating. BioRxiv https://biorxiv.org/cgi/content/short/2024.02.10.579740v1
- Latorraca NR, Sabaat S, Habrian C, Bleier J, Stanley C, Marqusee S, Isacoff EY. (2024). Domain coupling in activation of a family C GPCR. BioRxiv [Preprint]. 2024 Feb 29:2024.02.28.582567. doi: 10.1101/2024.02.28.582567. PMID: 38464305
Photo courtesy of Lawrence Berkeley National Laboratory, credit Roy Kaltschmidt.
Last Updated 2024-03-25