Gloria Brar
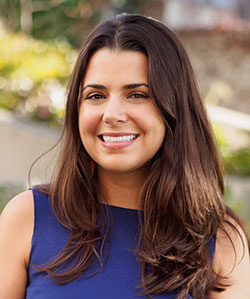
Professor of Cell Biology, Development and Physiology
Lab Homepage: http://www.brarlab.org/Research Interests
Unraveling the unconventional molecular circuitry underlying meiosis
(for more complete info, please check out www.brarlab.org)
Major motivating questions for the Brar lab include:
-How does gene expression regulation drive the dramatic changes that enable meiotic differentiation?
-What can gametogenesis teach us about how cells are constructed and deconstructed?
-Does the presence of many non-canonical gene regulatory features, even in the simple budding yeast, suggest that there is much that is not understood about gene expression, more generally?
Overview
Regulation of gene expression allows cells to grow, adapt to their environment, and differentiate into new forms. We know the key processes responsible—including transcription, translation, and protein degradation—but have a poor understanding of how they are naturally deployed and integrated to drive cellular change. The Brar lab studies the gene regulatory circuitry that underlies meiotic differentiation, the process that creates gametes. This conserved process involves dramatic and characteristic changes to cell morphology and function that reflect a series of unidirectional transitions in cell state. Meiosis fails frequently, with devastating consequences for fertility and health, but we do not understand the basis of these failures, due in part to gaps in our understanding of normal regulation. My lab has constructed an atlas of gene expression through meiosis, providing a framework to begin to explain its molecular control.
Our studies have also revealed new principles in gene regulation that may broadly apply to natural conditions of cellular change, and that have been missed by model-centric analyses. Canonical models for gene regulation are based on studies of individual genes and rational assumptions, such as a focus on cellular economy. They predate our ability to make genome-scale measurements. Such models tell us, for example, that proteins should be translated from Open Reading Frames (ORFs) that exceed 100 codons and that ORFs should initiate at AUG codons. Cases in which these rules do not fit have largely been treated as exceptions. Our studies of meiosis have shown that both the regions that are expressed and how they are regulated are not well explained by canonical gene regulatory rules. Systems-level studies, complemented with classical approaches, have allowed us to determine that unexpected patterns reflect substantial gaps in our understanding of how gene expression is naturally regulated. We are investigating the basis for these surprising findings, with the goal of discovering new gene regulatory features and explaining how they shape a meiotic cell. Our approach is to use integrated parallel global gene expression measurements and quantitative analytical frameworks, complemented with classical approaches, to gain fresh insight into eukaryotic gene regulatory circuitry.
The rules that govern how cells naturally use their genomic resources over time remain mysterious. Using the approaches described here, we aim to finally define them. We constructed the most comprehensive map of gene expression through a developmental process, including mRNA, translation, and protein measurements for many naturally synchronous stages of meiotic differentiation in budding yeast. We found strong temporal regulation of synthesis and degradation for nearly every gene in the genome, and these patterns were specific enough to enable accurate prediction of gene function. The highly parallel nature of the measurements enabled discovery of gene regulatory strategies that defy canonical models, including the widespread integration of translational and transcriptional control and the synthesis of thousands of non-canonical proteins.
Recent Studies
The development of techniques for measuring gene expression globally has greatly expanded our understanding of gene regulatory mechanisms in depth and scale. These methods finally allow scientists the opportunity to empirically revisit the canonical rules for gene expression in a systematic way and in diverse contexts. We can now quantify key intermediates and transitions in the canonical pathway of gene expression—from DNA to mRNA to protein—genome-wide. Our understanding of translation, in particular, has benefited immensely from the development of a tool—ribosome profiling—that enables its genome-wide measurement in vivo. Ribosome profiling, the deep sequencing of ribosome protected mRNA fragments, monitors protein synthesis with scale, speed, and accuracy rivaling approaches for mRNA measurement (Ingolia et al., Science, 2009; Ingolia et al., Nature Protocols, 2012; Brar and Weissman Nature Reviews Mol Cell Bio, 2015; Brar Cell 2016). We apply this method routinely, including to monitor translation in parallel with mRNA and protein levels at many time points. We have used such studies to generate a rich atlas of meiotic events and gene expression and the first high-resolution map of protein synthesis through a developmental program (Brar et al. Science, 2012; Cheng and Otto et al. Cell, 2018; Eisenberg et al. Cell Systems 2020).
Employing global measurements, including ribosome profiling, in parallel is a powerful approach that my lab has leveraged to paint a more complete picture of the gene regulatory circuitry underlying natural contexts of cellular change (Otto et al. Current Genetics, 2018). Our work has provided new and surprising insight into the natural eukaryotic regulation of gene expression and have revealed an unprecedented view of the molecular events underlying diverse aspects of meiotic biology and uncovered numerous new and dramatic instances of dynamic translational and transcriptional regulation through meiosis. These efforts have also yielded several fundamental surprises with broad significance, including the findings that: 1) Natural use of an unconventional gene regulatory mechanism based on timed toggling between two transcript isoforms of different translatability is common in meiosis and beyond (Cheng and Otto et al. Cell, 2018; Van Dalfsen et al. Developmental Cell 2018; Hollerer et al. G3 2019); 2)Protein degradation is a key driver of meiotic protein levels and, as a rule, protein complex members are synthesized imprecisely and matched by protein degradation during meiosis (Eisenberg and Higdon et al. Cell Reports, 2018); 3) Over 2000 novel short proteins are synthesized in a regulated manner during meiosis (Brar et al. Science, 2012; Ingolia et al. Cell Reports, 2014; Hollerer et al. Proteomics, 2018) 4) Yeast cells synthesize hundreds of alternative protein isoforms (Brar et al. Science, 2012; Eisenberg et al. Cell Systems 2020); and 5) Changes in the expression of the protein components of the ribosome are linked to characteristic gene expression signatures in meiosis and beyond (Cheng et al. Molecular Cell 2019; Cheng et al., Nucleic Acids Research 2019).
Current Projects
These discoveries have motivated several research directions in our lab that are focused on answering fundamental questions about gene regulation through meiosis.
Some questions that interest us are:
Find out more about our work in each of these areas by clicking the links embedded in the questions!
We love talking about our work and future projects so please feel free to contact us at gabrar@berkeley.edu ! And check out our lab website at www.brarlab.org or www.unal-and-brar-labs.org
Selected Publications
Higdon AL, Won NH, Brar GA. Truncated protein isoforms generate diversity of protein localization and function in yeast. Cell systems. 2024.
Powers EN, Reynaud K, Sousa C, Kuwayama N, Diamond P, Ingolia NT, Jovanovic M, Brar GA. Dbp1 is a low performance paralog of RNA helicase Ded1 that drives impaired translation and heat stress response. bioRxiv.2024.
Spiri S, Brar GA. Fix it, don’t trash it: Ribosome maintenance by chaperone-mediated repair of damaged subunits. Mol Cell 2023.
Vander Wende HM, Gopi M, Onyundo M, Medrano C, Adanlawo T, Brar GA. Meiotic resetting of the cellular Sod1 pool is driven by protein aggregation, degradation, and transient LUTI-mediated repression. J Cell Biol. 2023.
Powers EN, Chan C, Doron-Mandel E, Llacsahuanga Allcca L, Kim Kim J, Jovanovic M, Brar GA. Bidirectional promoter activity from expression cassettes can drive off-target repression of neighboring gene translation. Elife. 2022.
Sing TL, Brar GA, Ünal E. Gametogenesis: Exploring an Endogenous Rejuvenation Program to Understand Cellular Aging and Quality Control. Annu Rev Genet. 2022.
Barna M, Karbstein K, Tollervey D, Ruggero D, Brar G, Greer EL, Dinman JD. The promises and pitfalls of specialized ribosomes. Mol Cell. 2022.
Sing TL, Conlon K, Lu SH, Madrazo N, Morse K, Barker JC, Hollerer I, Brar GA, Sudmant PH, Ünal E.Meiotic cDNA libraries reveal gene truncations and mitochondrial proteins important for competitive fitness in Saccharomyces cerevisiae. Genetics. 2022.
Otto GM, Brar GA. Developmentally regulated selective autophagy determines ER inheritance by gametes. Autophagy. 2022.
Otto GM, Cheunkarndee T, Leslie J, Brar GA. Programmed cortical ER collapse drives selective ER degradation and inheritance in yeast meiosis. JCB. 2021.
Eisenberg AR, Higdon AL, Hollerer I, Fields AP, Jungreis I, Diamond PD, Kellis M, Jovanovic M, Brar GA.Translation Initiation Site Profiling Reveals Widespread Synthesis of Non-AUG-Initiated Protein Isoforms in Yeast. Cell Systems. 2020.
Cheng Z and Brar GA. Global translation inhibition yields condition-dependent de-repression of ribosome biogenesis mRNAs. Nucleic Acids Research. 2019.
Cheng Z, Mugler CF, Keskin A, Hodapp S, Chan LY, Weis K, Mertins P, Regev A, Jovanovic M, Brar GA. Small and Large Ribosomal Subunit Deficiencies Lead to Distinct Gene Expression Signatures that Reflect Cellular Growth Rate. Molecular Cell. 2019.
Eisenberg AR, Higdon A, Keskin A, Hodapp S, Jovanovic M, Brar GA. Precise Post-translational Tuning Occurs for Most Protein Complex Components during Meiosis. Cell Reports. 2018.
Van Dalfsen KM, Hodapp S, Keskin A, Otto GM, Berdan CA, Higdon A, Cheunkarndee T, Nomura DK, Jovanovic M, Brar GA. Global Proteome Remodeling during ER Stress Involves Hac1-Driven Expression of Long Undecoded Transcript Isoforms. Developmental Cell. 2018.
Otto GM and Brar GA. Seq-ing answers: uncovering the unexpected in global gene regulation. Curr Genetics. 2018
Hollerer I, Barker JC, Jorgenson V, Tresenrider A, Dugast-Darzacq C, Chan LY, Darzacq X, Tjian R, Ünal, E*, Brar GA*. Evidence for an Integrated Gene Repression Mechanism based on mRNA Isoform Toggling in Human Cells biorxiv 2018 (*equal contributions)
Guenther U-P, Weinberg DE, Zubradt MM, Tedeschi FA, Stawicki BN, Zagore LL, Brar GA, Licatalosi DD, Bartel DP, Weissman JS & Jankowsky E. The helicase Ded1p controls use of near-cognate translation initiation codons in 5′ UTRs. Nature. 2018.
Cheng Z*, Otto GM*, Powers EN, Keskin A, Mertins P, Carr SA, Jovanovic M, Brar GA. Pervasive, coordinated protein level changes driven by transcript isoform switching during meiosis. Cell. 2018 (*equal contributions)
Powers EN, Brar GA. m6A and eIF2α-p Team Up to Tackle ATF4 Translation during Stress. Mol Cell. 2018
Hollerer I, Higdon A, Brar GA. Strategies and Challenges in Identifying Function for Thousands of sORF-Encoded Peptides in Meiosis. Proteomics. 2017
Brar GA. Beyond the Triplet Code: Context Cues Transform Translation.Cell. Review. 2016 Dec 15;167(7):1681-1692
Brar GA and Weissman JS. Ribosome profiling reveals the what, when, where, and how of protein synthesis. Nature Reviews Molecular and Cell Biology. 2015 Nov;16(11):651-64.
Brar GA, Yassour M, Friedman N, Regev A, Ingolia NT, Weissman JS. High-Resolution View of the Yeast Meiotic Program Revealed by Ribosome Profiling. Science. Article. 2012 Feb 3;335(6068):552-7.
Ingolia NT, Brar GA, Stern-Ginossar N, Harris N, Talhouarne GJS, Jackson SE, Wills MR, and Weissman JS. Ribosome Profiling Reveals Pervasive Translation Outside of Annotated Protein-Coding Genes. Cell Reports (In Press).
Berchowitz LE, Gajadhar AS, van Werven FJ, De Rosa AA, Samoylova ML, Brar GA, Xu Y, Xiao C, Futcher B, Weissman JS, White FM, Amon A. A developmentally regulated translational control pathway establishes the meiotic chromosome segregation pattern. Genes Dev. 2013 Oct 1. PMID: 24115771.
Gilbert LA, Larson MH, Morsut L, Liu Z, Brar GA, Torres SE, Stern-Ginossar N, Onn Brandman, Whitehead EH, Doudna JA, Lim WA, Weissman JS, Qi LS. CRISPR-Mediated Modular RNA-Guided Regulation of Transcription in Eukaryotes. Cell. In press.
Thorburn RR, Gonzalez C, Brar GA, Christen S, Carlile TM, Ingolia NT, Sauer U, Weissman JS, Amon A. Aneuploid yeast strains exhibit defects in cell growth and passage through START. Mol Biol Cell. 2013 May; 24(9):1274-89.
Miller, M.P.*, Ünal, E.*, Brar, GA, Amon, A. (*equal contributions) Meiosis I chromosome segregation is established by inhibiting microtubule-kinetochore interactions in Prophase I. eLife. 2012 Dec 18; 1:e00117.
Ingolia NT, Brar GA, Rouskin S, McGeachy AM, Weissman JS. The ribosome profiling strategy for monitoring translation in vivo by deep sequencing of ribosome-protected mRNA fragments. Nature Protocols. 2012 Jul 26;7(8):1534-50.
Carvunis AR, Rolland T, Wapinski I, Calderwood MA, Yildirim MA, Simonis N, Charloteaux B, Hidalgo CA, Barbette J, Santhanam B, Brar GA, Weissman JS, Regev A, Thierry-Mieg N, Cusick ME, Vidal M. Proto-genes and de novo gene birth. Nature. 2012 Jul 19;487(7407):370-4.
Brar GA, Hochwagen A, Ee L, Amon A. The multiple roles of cohesin in meiotic chromosome morphogenesis and pairing. Mol Biol Cell. 2009 Feb;20(3):1030-47.
Brar GA and Amon A. Emerging roles for centromeres in meiosis I chromosome segregation. Nature Reviews Genetics. Review. 2008 Dec;9(12):899-910.
Brar GA, Kiburz BM, Zhang Y, Kim JE, White F, Amon A. Rec8 phosphorylation and recombination promote the step-wise loss of cohesins in meiosis. Nature. 2006 May 25;441(7092):532-6.
Hochwagen A, Tham WH, Brar GA, Amon A. The FK506 binding protein Fpr3 counteracts protein phosphatase 1 to maintain meiotic recombination checkpoint activity. Cell. 2005 Sep 23;122(6):861-73.
Photo credit: Mark Hanson at Mark Joseph Studios.
Last Updated 2024-08-26